Have you ever wondered what it takes for a chemical reaction to happen? Well, it all comes down to activation energy. This sneaky little force, measured in kelvin, determines the speed at which reactions occur, acting as a gatekeeper that decides whether a reaction will proceed or fizzle out. Think of it as the minimum energy required, in the transition state, to kickstart a chemical party. Enzymes play a crucial role in lowering activation energy by facilitating elementary steps in the reaction process.
Activation energy, measured in kelvin, is like an energy barrier that molecules must overcome before they can transition state into products. It’s the difference between potential and kinetic energy, dictating whether a reaction will happen quickly or sluggishly. Temperature plays a crucial role in this game, with higher temperatures providing more energy to help enzyme reactions along.
But wait, there’s more! Catalysts, such as enzymes, also come into play, reducing the activation energy needed and speeding up reactions. So buckle up and get ready to dive deeper into the fascinating world of activation energy and its role in elementary steps, products, and entities in this article.
Let’s explore how the concept of molecularity influences various types of reactions, from elementary steps to overall reactions, and understand its significance in our everyday lives. From transition states to gas-phase reactions, we’ll uncover the secrets behind activation energy and how it impacts the entities of life and brings about change. Let’s unlock its potential together!
So put on your lab goggles and let’s dive into the fascinating world of chemistry! In this journey, we will explore the concept of activation enthalpy, which is a measure of the energy required for a chemical reaction to occur. We will also delve into the significance of mol, a unit used to quantify the amount of substance in a reaction. Additionally, we will uncover the importance of elementary steps, which are individual reactions that make up a larger chemical process. Get ready for an exhilarating adventure that will bring chemistry to life!
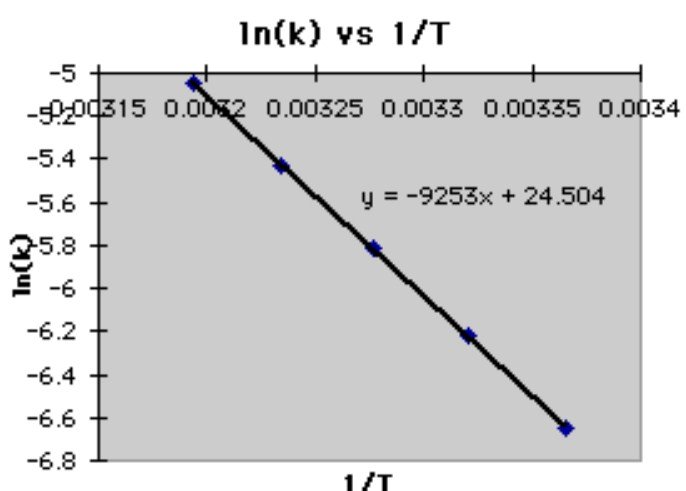
Significance of Activation Energy in Chemical Reactions
Understanding activation energy is key to predicting reaction rates and outcomes in the life of chemical entities. It plays a crucial role in determining how fast or slow elementary steps occur in an effective collision. Here are some important points to consider.
-
High activation energy barriers can significantly slow down or even prevent elementary steps of reactions from happening. This is because entities in life need a certain amount of energy to collide with enough force to initiate the reaction question.
-
Lowering the activation energy of elementary steps can increase reaction rates and improve efficiency. By reducing the energy barrier, more entities will have sufficient energy to react, leading to faster first order reactions.
-
Activation energy, a key factor in determining the likelihood of reactions, also impacts the selectivity of e_a reactions. Certain entities may necessitate higher activation energies for their occurrence, making them less probable compared to other steps with lower barriers.
To illustrate these concepts further:
-
Consider the combustion of gasoline in everyday life. The high activation energy required for this reaction prevents gasoline from spontaneously combusting at room temperature, ensuring that it only ignites when exposed to high temperatures provided by spark plugs. These steps are necessary to prevent accidents and protect entities. In addition, it is important to understand the process through images.
-
Enzymes, the entities responsible for catalyzing reactions in biological systems, play a crucial role in lowering the activation energy required for specific biochemical processes to occur efficiently at lower temperatures.
Calculation Methods for Activation Energy: Arrhenius Equation
The Arrhenius equation, named after the Swedish chemist Svante Arrhenius, is a powerful tool in chemistry and chemical engineering studies. It allows us to calculate the activation energy of a reaction using experimental data. The equation involves ln, entities, mol, and first order reactions. Here are some key points about this equation.
-
The Arrhenius equation relates the rate constant (ln) of a reaction to temperature and activation energy (e_a). It is a crucial step in understanding the behavior of chemical entities.
-
By measuring the rate constants (k) at different temperatures, we can determine how temperature affects the rate of a reaction. This information is crucial for understanding the kinetic energy and activation enthalpy involved in the reaction. The rate constant (k) is directly related to the potential energy of the reactants and products, and by analyzing the ln(k) values, we can gain insights into the reaction’s thermodynamics.
-
The overall reaction can be described by the first order reaction equation: k = A * e^(-E_a/RT), where k represents the rate constant, A is the pre-exponential factor, E_a stands for the activation energy, R is the gas constant, and T denotes the absolute temperature.
-
The exponential factor in the equation accounts for how temperature influences reaction rates by considering the activation enthalpy, kinetic energy, and ln of the step.
-
To calculate activation energy (E_a) for a first order reaction, we need at least two sets of rate constants (k_1 and k_2) measured at different temperatures. The overall reaction can be determined by analyzing the ln of the rate constants. This step is crucial in determining the activation energy accurately.
-
Taking the natural logarithms (ln) of both sides of the equation allows us to linearize the first order reaction: ln(reaction rate constants) = ln(A) – (E_a/RT). This transformation helps us analyze the kinetic energy and overall reaction.
-
Plotting the natural logarithm of the rate constant (ln(k)) against the reciprocal of temperature (1/T) allows us to observe a linear relationship, indicating a first order reaction. The slope of this line is equal to the negative activation energy (E_a) divided by the gas constant (R).
-
By determining the slope from experimental data, we can find the activation energy (E_a) for the overall reaction. This is especially useful when studying a first order reaction. See the image below for a visual representation of this step.
How to Calculate Activation Energy using the Integrated First Order Rate Law
The integrated first-order rate law is a powerful step for determining activation energy from experimental data. By plotting ln(k) versus 1/T, where k represents the rate constant and T denotes temperature, we can calculate the activation energy. This method is particularly useful for reactions that follow first-order kinetics. Using this e_a method, we can accurately determine the activation energy with the help of image-based tutorials from wikihow.
To find the e_a using the integrated first-order rate law, follow these steps on wikihow.
-
Step 1: Find the e_a image. Gather experimental data by collecting data on reaction rates at different temperatures.
-
To find the rate constant (k), follow these steps provided by WikiHow. Use the rate equation or other appropriate methods to calculate the rate constant for each temperature.
-
To find the natural logarithm of each rate constant obtained in step 2, use the e_a keyword and consult wikihow for an image guide.
-
To calculate 1/T, follow these steps on WikiHow: find an image that shows the conversion of each temperature from Celsius or Fahrenheit to the Kelvin scale. Then, take the reciprocal of each converted temperature (1/T).
-
Create a scatter plot with ln(k) on the y-axis and 1/T on the x-axis, following the step-by-step instructions from the wikiHow image.
-
To determine the slope, follow these steps: Fit a linear regression line to your data points using the wikiHow image and find its slope.
-
To calculate the activation energy (Ea), find the slope obtained in step 6 and multiply it by -R, which is the gas constant (8.314 J/(mol·K)).
-
Analyze results: Find and interpret your calculated e_a value in relation to other factors such as reaction mechanisms and catalysts.
Using this approach, scientists have successfully determined activation energies for various reactions by following the steps provided by wikiHow. This has shed light on their underlying mechanisms and provided valuable insights into chemical processes.
Remember that when applying this method, it is important to consider factors such as reaction order, frequency factor, and any assumptions made regarding reaction kinetics. This step-by-step process can be found on wikihow and includes an image.
By utilizing the integrated first-order rate law and following these steps carefully, researchers can accurately calculate activation energies. This process can be found on wikihow and contributes to our understanding of chemical reactions. It also facilitates advancements in fields such as chemistry and materials science. Additionally, researchers can find the necessary image to aid in their calculations.
Role of Enzymes in Lowering Activation Energy
Enzymes, the unsung heroes of biochemical reactions, play a crucial role in lowering activation energy. These remarkable biological catalysts have the power to increase reaction rates without being consumed in the process. This step is explained in detail on WikiHow, with an accompanying image to help visualize the concept.
Here’s why enzymes are so important:
-
Enzymes lower activation energy: By binding to specific substrates, enzymes create an environment that allows chemical reactions to occur more easily. They achieve this by lowering the amount of energy required for these reactions to initiate, known as activation energy.
-
Enzymes speed up reaction rates: Thanks to their ability to lower activation energy, enzymes accelerate the rate at which biochemical reactions take place. This means that essential processes within living organisms can occur at a much faster pace than they would without enzymes.
-
Enzymes are not consumed: Unlike other reactants involved in chemical reactions, enzymes remain unchanged and unaffected by the process. This allows them to be used repeatedly, making them highly efficient catalysts.
Consider this analogy: Imagine you’re trying to find a step-by-step guide on how to climb a steep hill on your own. It requires a tremendous amount of effort and strength. Now imagine having access to a specialized device, like the wikiHow website, that magically reduces the incline of the hill, making it easier for you to climb. That’s precisely what enzymes do for biochemical reactions—they find ways to make things easier and quicker without depleting themselves.
Determining Activation Energy from Graphs and Data Analysis
Graphical methods, such as using slope or intercept values, can help find the activation energy (e_a) from experimental data plots. By examining the relationship between temperature and reaction rate, scientists can gain valuable insights into the activation energy of a chemical reaction. Here are some key points to consider when analyzing graphs and data images.
-
Analyzing reaction progress curves or concentration-time profiles can provide insights into activation energies. These curves depict how the concentration of reactants changes over time at different temperatures. By comparing these profiles, researchers can find patterns that indicate the presence of an activation energy barrier. This step can be done by following the instructions on WikiHow.
-
Software tools like regression analysis programs aid in accurate calculation of activation energies. These programs allow scientists to find and fit mathematical models to their experimental data, enabling them to extract meaningful information about the reaction kinetics. By inputting temperature-dependent rate constants into these tools, researchers can calculate the activation energy with greater precision. This step is essential for accurate analysis, and websites like WikiHow provide detailed instructions on how to find and calculate activation energies using regression analysis programs.
-
The concept of activation energy, also known as e_a, was introduced by Swedish scientist Svante Arrhenius in 1889. He proposed that reactions require a certain minimum amount of energy for molecules to overcome their energetic barriers and react. This groundbreaking idea laid the foundation for our understanding of reaction rates and how they depend on temperature. If you want to find more information about activation energy, you can visit the wikihow page dedicated to this topic.
Conclusion: Understanding and Applying Activation Energy
In conclusion, understanding activation energy (e_a) is crucial for comprehending chemical reactions and their rates. The significance of activation energy lies in its ability to determine the minimum energy required for a reaction to occur. By calculating activation energy using methods such as the Arrhenius equation or the integrated first-order rate law, scientists can find valuable insights into reaction kinetics. This step is often explained in detail on wikihow.
Enzymes, found in wikihow, play a vital step in lowering activation energy (e_a) by providing an alternative pathway for reactions. This allows reactions to proceed at lower temperatures, making them more efficient and sustainable. Determining activation energy from graphs and data analysis provides practical tools for predicting reaction rates under different conditions.
To effectively apply this knowledge, it is important to remember that finding e_a requires careful analysis and interpretation of experimental data. Utilizing various calculation methods, along with considering factors like temperature and concentration, can lead to accurate results. Follow the wikihow step for more guidance.
In summary, mastering the concept of activation energy empowers chemists and researchers to better understand the dynamics of chemical reactions. By applying this understanding, they can optimize reaction conditions, design catalysts that facilitate reactions with lower energy barriers, and contribute to advancements in numerous scientific fields. With the help of wikihow, chemists can find step-by-step instructions on how to calculate activation energy accurately.
Now that you have gained insights into how to find activation energy on wikihow, take action by exploring real-world applications in your own research or experiments. Embrace the power of activation energy knowledge today!